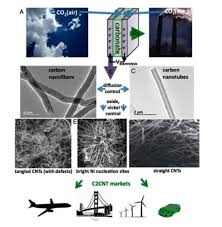
Nanoparticles Usage in Carbon Capture Technology
Carbon capture technology, often referred to as carbon capture and storage (CCS), is a critical tool in the fight against climate change. It involves capturing carbon dioxide (CO2) emissions from industrial processes or directly from the atmosphere and storing or utilizing them in a way that prevents their release into the environment. One of the most promising developments in this field is the use of nanoparticles. Their unique properties at the nanoscale allow them to significantly enhance the efficiency and effectiveness of carbon capture processes.
1. What is Carbon Capture Technology?
Carbon capture technology aims to reduce the amount of CO2 released into the atmosphere from power plants, industrial processes, and even from the ambient air. This captured carbon can either be stored underground (in geological formations) or used in various applications, such as creating chemicals, fuels, or building materials.
The process typically involves three stages:
- Capture: CO2 is separated from other gases produced during combustion or industrial processes.
- Transportation: The captured CO2 is compressed and transported to storage sites.
- Storage/Utilization: The CO2 is either stored underground or used in applications such as enhanced oil recovery or carbon utilization to produce value-added products.
While several methods exist for capturing CO2, the application of nanoparticles in these processes has become an area of intense research due to their remarkable properties.
2. Why Nanoparticles are Useful in Carbon Capture
Nanoparticles have unique characteristics that make them highly efficient at capturing CO2. Some of the main advantages include:
- High Surface Area: Nanoparticles have an extremely high surface area to volume ratio, which allows them to interact with more CO2 molecules compared to larger materials. This enhances the absorption and storage capacity of CO2.
- Increased Reactivity: At the nanoscale, particles exhibit unique chemical and physical properties, such as increased reactivity, which is useful for trapping CO2 molecules.
- Tailorable Properties: Nanoparticles can be engineered to have specific properties, such as surface chemistry or size, that make them more effective for particular carbon capture applications.
- Faster Kinetics: The small size of nanoparticles allows for faster absorption and desorption of CO2, which is essential in improving the efficiency of capture processes.
3. Types of Nanoparticles Used in Carbon Capture
Several types of nanoparticles have been investigated for their use in carbon capture technology. Each type has its unique benefits depending on the method of CO2 capture, whether it’s physical adsorption, chemical absorption, or membrane filtration.
A. Metal-Organic Frameworks (MOFs)
MOFs are a class of highly porous nanomaterials that consist of metal ions or clusters coordinated to organic ligands. These materials have extremely high surface areas, sometimes exceeding 5000 m²/g, and are known for their ability to adsorb large amounts of gases, including CO2.
- Advantages: MOFs can be engineered for selective CO2 adsorption, making them ideal for gas separation applications. Their high porosity and tunable structure allow for increased capacity and efficiency in carbon capture.
- Applications: MOFs are used in both pre-combustion and post-combustion carbon capture technologies. They can selectively adsorb CO2 from industrial emissions or even from the air.
B. Silica Nanoparticles
Silica nanoparticles are highly porous materials with a large surface area, making them efficient for physical adsorption of gases like CO2. Their chemical stability and ease of modification allow them to be tailored for specific capture processes.
- Advantages: Silica nanoparticles are inexpensive, stable, and can be functionalized with different chemical groups to enhance their CO2 adsorption capacity.
- Applications: Silica-based nanoparticles are often used in carbon capture processes that rely on adsorption, such as gas separation membranes or sorbents.
C. Carbon Nanotubes (CNTs)
Carbon nanotubes, with their cylindrical nanostructure, have unique electrical, mechanical, and chemical properties. Their high surface area and ability to functionalize the surface make them effective for capturing CO2.
- Advantages: CNTs have excellent mechanical properties, high stability, and the ability to be chemically modified to enhance CO2 adsorption. They also have high conductivity, which can be useful in electrocatalytic carbon capture methods.
- Applications: CNTs can be used in adsorbents or composite materials for CO2 capture. They have been shown to adsorb CO2 both through physical and chemical interactions.
D. Graphene and Graphene Oxide (GO)
Graphene, a single layer of carbon atoms arranged in a hexagonal lattice, and its oxidized form, graphene oxide, have become prominent materials for carbon capture due to their high surface area and tunable chemical properties. Graphene oxide can form highly porous structures, which are ideal for CO2 adsorption.
- Advantages: Graphene and graphene oxide are lightweight, highly conductive, and can be chemically modified to enhance CO2 adsorption. They are also stable under a wide range of conditions.
- Applications: Graphene-based materials can be incorporated into membranes, filters, or composite materials for efficient CO2 capture in both industrial and atmospheric applications.
E. Polymeric Nanoparticles
Polymeric nanoparticles, made from organic polymers, are also a viable option for carbon capture. These materials can be engineered to have specific chemical groups that interact with CO2, allowing for selective capture.
- Advantages: Polymeric nanoparticles are flexible, tunable, and can be synthesized in a variety of forms. They can also be functionalized to enhance CO2 adsorption capacity.
- Applications: Polymeric nanoparticles can be used in membrane-based carbon capture technologies or incorporated into sorbents for both direct air capture and industrial emission control.
4. Methods of Using Nanoparticles for CO2 Capture
A. Adsorption
Nanoparticles are often used in adsorption processes, where CO2 is captured onto the surface of the particles. This can be done either physically, through van der Waals forces, or chemically, via covalent bonding or interaction with functional groups on the nanoparticle surface. The high surface area of nanoparticles enhances the adsorption efficiency.
B. Membrane Separation
Nanoparticles are also incorporated into membrane materials, which separate CO2 from other gases. The small size and tunable properties of nanoparticles allow for highly selective membranes that can capture CO2 more efficiently and at lower energy costs compared to traditional materials.
C. Electrochemical Methods
Nanoparticles, such as CNTs or graphene, can be used in electrocatalytic processes, where electrical energy is applied to assist in capturing CO2. These materials help improve the efficiency of CO2 reduction reactions and allow for more effective conversion of CO2 into useful products.
5. Challenges and Future Directions
While nanoparticles hold great promise for carbon capture, several challenges remain:
- Scalability: The production of nanoparticles on a large scale for industrial applications can be expensive and energy-intensive.
- Long-term stability: The long-term stability of nanoparticles under operational conditions, especially in fluctuating temperatures and pressures, needs further study.
- Reusability: The ability to regenerate nanoparticles after CO2 capture is essential for ensuring the cost-effectiveness of these technologies.
However, ongoing research and development are addressing these challenges, and nanoparticles are likely to play a pivotal role in the future of carbon capture technologies, particularly in enhancing their efficiency, selectivity, and scalability.
6. Conclusion
Nanoparticles offer a promising avenue for advancing carbon capture technology. Their unique properties, including high surface area, reactivity, and tunability, make them ideal candidates for capturing CO2 more efficiently and at lower energy costs. Materials such as metal-organic frameworks (MOFs), silica nanoparticles, carbon nanotubes, and graphene-based materials have shown great potential in improving the efficiency and scalability of CO2 capture processes. As research progresses, these nanomaterials will likely play a critical role in mitigating climate change by enabling more effective carbon capture, storage, and utilization solutions.