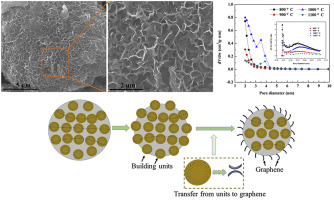
Graphene Microbeads and Their Applications: A Revolutionary Nanomaterial
Graphene, a single layer of carbon atoms arranged in a two-dimensional honeycomb lattice, has earned its place as one of the most groundbreaking materials of the 21st century. Since its discovery, graphene’s extraordinary electrical, thermal, mechanical, and optical properties have sparked extensive research into its applications across various industries. One of the exciting developments in graphene technology is the creation of graphene microbeads — small, spherical particles of graphene that maintain many of the remarkable properties of graphene while offering new opportunities for industrial applications.
In this article, we will explore what graphene microbeads are, their properties, how they are synthesized, and the diverse applications they are contributing to, ranging from electronics and energy storage to biomedicine and environmental remediation.
What Are Graphene Microbeads?
Graphene microbeads are spherical nanoparticles composed of graphene sheets or graphene oxide that are aggregated or encapsulated into microscale beads (typically ranging in size from a few micrometers to hundreds of micrometers). These microbeads retain the intrinsic properties of graphene, such as high electrical conductivity, mechanical strength, and thermal stability, but with the added benefit of their spherical form factor. This unique structure makes them easier to handle, process, and integrate into a variety of applications.
Graphene microbeads can be produced in several forms, including pure graphene, graphene oxide, or reduced graphene oxide, depending on the desired characteristics for specific uses. The surface area and functionalization of the graphene microbeads can be adjusted to enhance specific properties like solubility, dispersion, and biocompatibility.
Properties of Graphene Microbeads
Graphene microbeads inherit many of the outstanding properties of graphene in their microbead form. These properties make them valuable for numerous applications, particularly in areas where high performance, lightweight, and efficient materials are required. The main properties of graphene microbeads include:
1. High Electrical Conductivity
- Like graphene, graphene microbeads exhibit excellent electrical conductivity, which makes them useful for energy storage, sensors, and electronic devices. Their high conductivity also makes them ideal candidates for supercapacitors and batteries.
2. Mechanical Strength and Durability
- Graphene microbeads retain the mechanical properties of graphene, which is renowned for being one of the strongest materials known. This makes them useful in applications requiring high strength-to-weight ratios or enhanced durability, such as in composites and coatings.
3. High Surface Area
- Despite their spherical form, graphene microbeads still possess a high surface area, which is beneficial for adsorption and catalysis. A large surface area increases the effectiveness of the material in applications like catalytic converters, gas storage, and water filtration.
4. Thermal Stability
- Graphene microbeads exhibit high thermal conductivity and stability, allowing them to perform well in high-temperature applications. Their ability to conduct heat efficiently makes them suitable for heat management systems and thermal interface materials.
5. Biocompatibility and Functionalization
- Graphene microbeads can be functionalized with different chemical groups to make them more biocompatible and soluble in biological environments. This allows their use in biomedical and drug delivery applications.
Synthesis of Graphene Microbeads
The synthesis of graphene microbeads generally involves the aggregation or encapsulation of graphene sheets or graphene oxide into microscale spherical structures. Some of the commonly used methods for producing graphene microbeads include:
1. Sol-Gel Method
- The sol-gel process is often used to synthesize graphene oxide microbeads. In this method, graphene oxide dispersions are mixed with sol-gel precursors, and the formation of gel-like spheres is induced by changes in temperature or the addition of certain chemicals. These spheres can be subsequently reduced to graphene microbeads.
2. Electrostatic Assembly
- Electrostatic assembly involves the use of electrostatic forces to create graphene microbeads from graphene oxide sheets. A graphene oxide suspension is subjected to an electric field, which causes the particles to aggregate into spherical shapes. The process can be controlled to produce microbeads with precise sizes.
3. Hydrothermal Synthesis
- Hydrothermal synthesis is a popular method for creating graphene oxide or reduced graphene oxide microbeads. This technique involves the chemical reduction of graphene oxide in the presence of a reducing agent under high temperature and pressure conditions, resulting in the formation of spherical graphene microbeads.
4. Emulsion Polymerization
- In emulsion polymerization, graphene oxide is mixed with monomers to create graphene-based composite microbeads. The polymerization process occurs in an emulsion system, leading to the formation of graphene microbeads with improved stability and functionality.
5. Template-assisted Methods
- Template-assisted methods involve using a template or mold to form graphene microbeads. Graphene oxide or graphene dispersions are added to a mold, and after drying or hardening, the template is removed to leave behind graphene microbeads.
Applications of Graphene Microbeads
Graphene microbeads have a broad range of applications across various industries, from electronics and energy storage to medicine and environmental protection. Some of the most promising applications include:
1. Energy Storage
- Supercapacitors and Batteries: Graphene microbeads are highly effective in energy storage devices, such as supercapacitors and lithium-ion batteries. Their high conductivity, large surface area, and mechanical strength make them ideal for enhancing the charge/discharge cycles and capacity of these devices.
- Fuel Cells: Graphene microbeads are also being studied for use in fuel cells due to their excellent conductivity and electrocatalytic properties.
2. Environmental Remediation
- Water Purification: Graphene microbeads can be used in water filtration systems to remove heavy metals, organic contaminants, and other pollutants from water. Their high surface area and adsorption capabilities make them effective in purifying water.
- Oil Spill Cleanup: Due to their hydrophobic nature and high adsorption capacity, graphene microbeads can be used in the cleanup of oil spills and other environmental disasters, absorbing large quantities of oily contaminants from water surfaces.
3. Biomedical Applications
- Drug Delivery: Functionalized graphene microbeads can be used as carriers for drug delivery systems. Their biocompatibility and large surface area enable the efficient loading and controlled release of therapeutic agents, making them ideal for targeted drug delivery and cancer treatment.
- Biosensors: Graphene microbeads can be utilized in the development of biosensors for detecting biomolecules, pathogens, and diseases. Their high conductivity and ability to functionalize with biomolecules make them effective in diagnostic applications.
4. Electronics and Sensors
- Flexible Electronics: Graphene microbeads can be incorporated into flexible electronics due to their excellent electrical properties and mechanical flexibility. They are used in transparent conductors for smart displays and wearable electronics.
- Gas Sensors: Graphene microbeads are highly sensitive to gases like CO₂, NO₂, and NH₃. Their use in gas sensors can help monitor air quality, industrial emissions, and environmental pollution.
5. Coatings and Composite Materials
- Protective Coatings: Graphene microbeads can be used to enhance the properties of coatings for anti-corrosion, anti-wear, and anti-UV protection. When incorporated into coatings, graphene microbeads improve durability, adhesion, and thermal stability.
- Polymer Composites: Graphene microbeads can be integrated into polymer composites to enhance their mechanical properties, making them suitable for a variety of applications in aerospace, automotive, and construction.
Challenges and Future Outlook
While graphene microbeads hold great promise, there are several challenges that need to be addressed before they can achieve widespread adoption:
- Scalability: Producing high-quality graphene microbeads in large quantities at an affordable cost remains a challenge. Researchers are working on improving synthesis methods to achieve mass production.
- Toxicity and Environmental Impact: The potential toxicity of graphene and its impact on the environment need to be thoroughly assessed. Safe handling, disposal, and biocompatibility studies will be crucial for ensuring the responsible use of graphene microbeads.
- Functionalization and Stability: Tailoring the surface properties of graphene microbeads for specific applications (e.g., biomedical or environmental) remains a key challenge. The stability of these microbeads in different environments needs to be optimized.
Conclusion
Graphene microbeads represent a versatile and powerful class of nanomaterials with numerous applications in energy storage, environmental protection, biomedical fields, and electronics. Their unique properties, including high electrical conductivity, mechanical strength, and large surface area, make them ideal for a wide range of applications, from supercapacitors to biosensors and smart coatings. With ongoing research focused on overcoming challenges related to scalability, toxicity, and functionalization, graphene microbeads are poised to revolutionize multiple industries, paving the way for advanced technologies and sustainable solutions in the future.