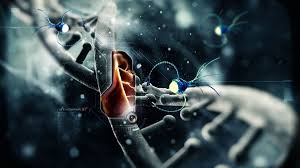
From Complexity to Functionality: Molecular Nanotechnology and Nanosystems
Molecular nanotechnology and nanosystems have become revolutionary fields with the potential to transform various industries, from medicine to electronics. At the heart of these innovations is the ability to manipulate and design materials at the molecular and atomic levels. By understanding the complexity of molecular interactions and engineering nanoscale systems, scientists and engineers are paving the way for new materials and devices that exhibit unprecedented functionalities. This article delves into the journey from complexity to functionality in molecular nanotechnology and nanosystems, highlighting their principles, applications, challenges, and future directions.
1. Introduction to Molecular Nanotechnology and Nanosystems
Molecular nanotechnology refers to the design, manipulation, and fabrication of functional systems at the molecular scale, typically ranging from 1 to 100 nanometers. Nanosystems are engineered structures that integrate multiple molecular components to perform specific tasks, often mimicking or improving upon natural systems.
Molecular nanotechnology is distinct because it focuses on building materials and devices atom by atom or molecule by molecule. This contrasts with traditional top-down approaches, such as lithography, where materials are sculpted from bulk substances. Instead, molecular nanotechnology seeks to harness the inherent properties of molecules and atoms to achieve functionality that would be impossible or inefficient with larger, bulk materials.
2. The Complexity of Molecular Nanotechnology
At the core of molecular nanotechnology lies an understanding of complexity—how simple molecular components can interact and assemble to form larger, functional systems. This complexity emerges from the following factors:
- Molecular Interactions: The interactions between molecules, including covalent bonding, hydrogen bonding, van der Waals forces, and ionic interactions, govern the behavior of nanosystems. These interactions are often subtle and complex but play a critical role in the design of nanomaterials.
- Self-Assembly: One of the most fascinating aspects of molecular nanotechnology is self-assembly, where molecules spontaneously organize themselves into desired structures without external guidance. This occurs due to the specific properties of the molecules involved, such as hydrophobicity, electrostatic interactions, or geometric compatibility.
- Molecular Machines and Motors: Molecular machines are nanoscale devices that perform specific tasks, such as moving in response to external stimuli or carrying out chemical reactions. Examples include molecular motors that convert chemical energy into mechanical motion, similar to biological machines like ATP synthase in cells.
- Complexity in Functionality: The complexity arises when these molecular components combine to perform sophisticated functions. Whether in the form of drug delivery systems or molecular sensors, the ability of nanosystems to perform multiple tasks simultaneously or under specific conditions is what makes molecular nanotechnology so promising.
3. From Complexity to Functionality: Engineering Nanosystems
Turning molecular complexity into functional nanosystems requires careful design and engineering. Researchers rely on various techniques to ensure that the systems they create can perform specific tasks reliably:
- Molecular Design and Simulation: Advanced computational techniques, such as molecular dynamics simulations, allow researchers to predict how molecules will behave when assembled into larger structures. This helps optimize design and predict possible outcomes before physical experiments are conducted.
- Nanofabrication Techniques: Methods such as DNA origami, where DNA strands are folded into specific shapes, or top-down fabrication techniques, are employed to construct nanosystems with precise control. These methods help build more intricate and functional devices that can be used in real-world applications.
- Interfacing with Biological Systems: The interface between nanosystems and biological systems is a critical challenge in the field. For example, in drug delivery, it’s necessary for the nanomaterials to interact with human cells in specific ways, ensuring biocompatibility and avoiding toxicity. Functionalities such as targeting specific cells or releasing drugs in a controlled manner require a detailed understanding of both molecular biology and material science.
4. Applications of Molecular Nanotechnology and Nanosystems
Molecular nanotechnology and nanosystems have vast potential in numerous fields. Some key applications include:
A. Medicine and Healthcare
- Drug Delivery Systems: Nanomaterials can be designed to deliver drugs in a targeted and controlled manner, minimizing side effects and maximizing therapeutic efficacy. For example, nanoparticles can be engineered to deliver chemotherapy directly to cancer cells, leaving healthy cells unharmed.
- Nanosensors for Diagnostics: Nanosensors can detect disease biomarkers with high sensitivity, enabling early detection of diseases such as cancer or infections at the molecular level. These sensors can be integrated into wearable devices for continuous monitoring of health parameters.
- Tissue Engineering: Nanosystems can aid in creating synthetic tissues and organs by guiding the growth of cells on nanoscale scaffolds. These technologies are pivotal in regenerative medicine, potentially offering solutions for organ transplants and healing damaged tissues.
B. Electronics and Computing
- Molecular Electronics: Nanosystems are revolutionizing electronics by enabling the development of smaller, faster, and more efficient devices. Molecular transistors, for example, could provide a pathway to ultra-dense, low-power electronics that surpass the limitations of traditional silicon-based systems.
- Quantum Computing: Molecular nanotechnology is expected to play a critical role in the development of quantum computers. Nanosystems can be designed to exploit quantum mechanical phenomena like superposition and entanglement to perform complex calculations far beyond the capabilities of classical computers.
C. Environmental and Energy Applications
- Energy Storage and Conversion: Nanosystems can be used in developing more efficient batteries, fuel cells, and solar cells by improving energy storage capacity and reducing energy loss. Nanomaterials, like carbon nanotubes or graphene, are being explored for their superior electrical conductivity and ability to enhance the performance of energy devices.
- Pollution Control: Nanosystems can be designed to remove pollutants from water or air. For instance, nanoparticles are used in water filtration systems, where they can selectively absorb harmful substances or break them down into less toxic compounds.
5. Challenges and Future Directions
While the potential of molecular nanotechnology and nanosystems is vast, several challenges remain:
- Scalability: Moving from the laboratory-scale production of nanosystems to industrial-scale manufacturing is a significant hurdle. The precision required to build nanosystems atom by atom or molecule by molecule can be difficult to achieve at larger scales.
- Integration with Existing Technologies: Successfully integrating molecular nanosystems with current technologies and systems, particularly in fields like electronics and healthcare, requires overcoming compatibility and standardization challenges.
- Ethical and Environmental Concerns: As with any emerging technology, molecular nanotechnology raises ethical concerns, including issues of privacy, safety, and environmental impact. Responsible research and development practices must be followed to ensure that these technologies are used safely and sustainably.
6. Conclusion
Molecular nanotechnology and nanosystems represent an exciting frontier in science and engineering, where complexity transforms into functionality. By harnessing the unique properties of materials at the nanoscale, researchers are creating new devices and systems that were once thought to be impossible. While challenges remain in terms of scalability, integration, and safety, the future of molecular nanotechnology holds enormous promise, offering transformative solutions across a wide array of industries—from healthcare to energy and beyond. As technology continues to evolve, it is clear that molecular nanotechnology will play a central role in shaping the future of innovation and functionality.